NIC Excellence Project 2023/2
Collective Dynamics of Intelligent Microswimmers
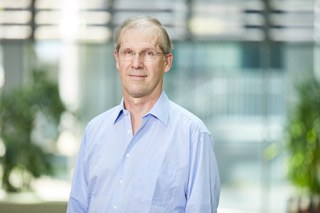
John von Neumann Excellence Project 2023/2
Prof. Gerhard Gompper (Universität Köln and Forschungszentrum Jülich)
Sensing of the environment and information processing, combined with motility, is a fundamental characteristic of life, from the largest animals to the smallest single-cell organisms, and is responsible for the astonishing success for survival of individuals and groups on all scales. The adaptive self-steering gives rise to fascinating phenomena, ranging from large-scale collective behaviors denoted as swarming, as observed in mammalian herds, flocks of birds, school of fish, or even cell layers and tissues, to the formation of bacterial biofilms. A detailed understanding of microbial transport ultimately opens avenues for control of biological systems and the design of novel artificial nano- and micromachines (“microbots”). In order to achieve a similar spectrum of functionality as in biological microorganisms, current concepts of synthetic active particles need to be augmented with sensing and information-processing capacities. This will establish a novel class of intelligent active systems with extraordinary properties, such as self-healing, self-steering, autonomous delivery, swarm cohesion, and collective reactions to external signals.
Many self-propelled agents operate in an aqueous environment. Here, the fluid is required for their propulsion, but also implies additional fundamental interparticle interactions, such as thermal noise and flow-mediated forces. In combination with other input signals, such hydrodynamic effects lead to a more complex dynamical behavior. As a long-term vision, we aim to establish a generic model of an intelligent microbot embedded in a fluid and to analyze the emergent collective behaviors, which will ultimately serve as a framework to elucidate the interplay of the various interactions, sensing, and steering mechanisms of microswimmers.
On the modeling side, we employ a particle-based mesoscale hydrodynamics simulation technique, called Multi-Particle Collision Dynamics (MPC), for the fluid dynamics. In this approach, fluid particles are sorted into small boxes, which define the region of local momentum exchange, which has the computational advantage that no distances between fluid particles have to be calculated. The self-propelled microswimmers are described as “squirmers”, spherical objects with a prescribed velocity field on their surfaces. Two important classes of squirmers have to be distinguished: pullers with propulsion in the front (like breaststroke swimmer), and pushers, with the propulsion in the rear (like ship with outboard motor). The swimmers sense the orientation of neighboring swimmers, and steer their own motion such as to align with the majority of neighbors. Steering is achieved by an additional, non-axisymmetric surface slip velocity with controllable magnitude, which is called maneuverability – an important parameter of the model.
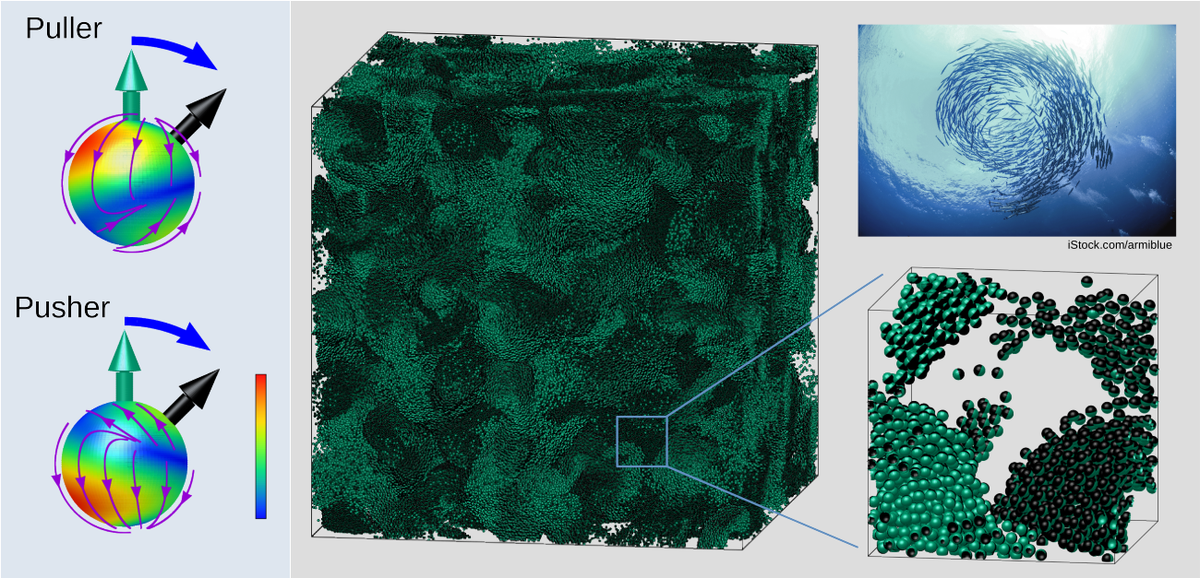
In previous work, we have studied the pursuit of a self-propelled target/prey by a self-steering pursuer/predator in a fluid environment. Here, we found, inter alia, that hydrodynamic effects may enhance or reduce the pursuit performance depending on the swimming mechanism (puller vs. pusher). Moreover, self-steering of a pursuer and its hydrodynamic flow field also affect the target motion, which for microbots can be utilized to guide the target to a preselected location.
Preliminary studies of ensembles of swimmers with alignment interactions exhibit pronounced self-organization, like clustering and formation of swirls, with a strong dependence on parameters like the force-dipole strength of the squirmer (which measures the imbalance between front and rear pushing) and the maneuverability. Our simulations indicate that the interplay of hydrodynamic and self-steering effects gives rise to a rich dynamical behavior beyond a simple order-disorder transition. Our large-scale hydrodynamic simulation approach on massively parallel, GPU-based supercomputers like JUWELS allows the exploration and unraveling of the complex dynamical behavior of swimmer swarms, from microorganisms to microbots.